"What is
Physics Good For?"
Extra credit is available at the end of this
page. Please respond before 9 AM, Monday, November
6th, 2000.

"Seeing"
Atoms
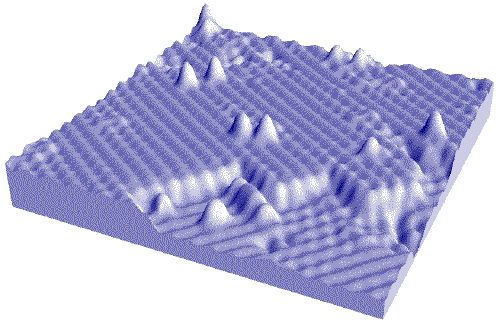 |
STM image of the surface
of a silicon crystal, courtesy of Dr. B.
Swartzentruber, Sandia National Labs |
Physics is also good for physics, ... and
chemistry, biology, geology, and other fields in
science and enginnering. This week, "What Is
Physics Good For?" departs from the subject we are
studying in the classroom, and dives into an area
of physics research that has seen huge gains in
recent years, and has produced profound effects in
many other areas: scanned probe microscopy.
Not very long ago, this was a truism of modern
science: "we know atoms exist, but we can never see
them." That was before 1982. In that year, Gerd Binnig and Heinrich Rohrer
published their first work on a new device known as
the Scanning Tunneling Microscope, or STM for
short. In 1986, they were awarded the Nobel Prize
in physics for their work.
This article will describe this breakthrough
that has at last allowed us to "see" atoms. I have
kept the term "see" in quotes, because the
microscope in question does not operate by bouncing
light off a sample and creating a magnified image.
Rather, the STM operates by taking advantage of
quantum tunneling to measure the the shape of the
electron "cloud" at the surface of a material. That
is, it makes a contour map of the surface, where
the contours follow lines of constant electron
density. Some people will argue that this is not
really "seeing", but there can be little doubt that
the peaks and valleys on many STM images represent
individual atoms and the spaces between them.
How does this amazing device work? The key is in
quantum tunneling. Tunneling is an essential
feature of quantum mechanics, that we can
understand in terms of Heisenberg's uncertainty
principle. In one form, the uncertainty principle
says
We can interpret this to mean that the energy of a
particle can increase for a short period of time,
even if the energy does not come from the
particle's surroundings. That is, for short periods
of time, energy is not conserved. The implications
of this notion are astounding. In the case of
tunneling, the particle briefly "borrows" an extra
bit of energy from nowhere, uses it to travel
through a barrier and then gives the enrgy back
after it is done. Once the process is done, the
particle has it's original energy back, but it now
is on the other side of the barrier.
Here is an analogy. A marble is placed in a cereal
bowl and given enough of a push to roll half way up
the side. If there is little friction, the ball can
roll back and forth for a while, but it will never
have enough energy to roll all the way up and over
the wall. It cannot overcome the barrier. However,
for a subatomic particle, the situation is very
different. The particle's energy is tiny, and so
are the barriers it faces. There is a reasonable
chance that a subatomic particle can pass over a
barrier and escape. Once it is out, it doesn't need
the enrgy anymore.
This is how the scanning tunneling microscope
works. In the STM, a very sharp metal tip (usually
tungsten) is held near the surface of a conducting
sample. A small valtage (usually 1.5 to 3 V) is
placed between the tip and the sample. Now, the
electrons in the tip would like to flow to the
sample. If the tip touches the sample current will
flow easily, if the tip is far away, the electrons
cannot overcome the barrier and "jump" the gap.
However, if the distance is just right, the
electrons can tunnel out of the tip and get into
the sample. This process is illustrated in the
diagrams.
What makes this process truly wonderful (besides
the fact that it can happen at all) is the
tunneling current depends very sensitively on the
size of the gap. If we move the tip laterally over
the sample, the current changes strongly in
response to variations in the surface height. This
is known as constant height mode. What is actually
even better is constant current mode. In this
operation, the tip is moved laterally, and as it
moves its height is adjusted in order to keep the
tunneling current constant. As this process occurs,
the height adjustments are recorded. The tip
literally follows the contours of the sample.
It would be a shame to leave you with the
impression that STM is a scientific curiosity with
no purpose beyond making pretty pictures. On the
contrary, STM is a powerful tool for learning new
and useful information about the physics and
chemistry of surfaces. One example is in the
understanding of how films of materials grow. Many
important technologies (semiconductors and magnetic
storage come to mind) depend on devices that are
made of thin films of material (silicon and
magnetic alloys in those two examples). The
precision of the devices depends crucially on the
precision with which the films can be grown. STM
has helped to solve many problems in our
understanding of film growth.
The figure to the left shows an example
(courtesy of Dr. J. Stroscio of the National Institute for Standards and
Technology). In these images we do not see the
individual atoms laterally, but we do see single
atom changes in height. The brightness represents
the height of the surface, brighter areas are
higher than dark. Thus, the film on the left (a)
has grown as many small islands, and there are at
least three separate layers showing. On the other
hand, the film on the right (b) is growing in a
"layer-by-layer" mode. This is desirable because
the resulting film will be flatter and have fewer
defects. Furthermore, if it is necessary to grow
another film on top, the next film will have a
better surface to start from, and there may be less
intermixing. What allowed the improvement? The only
difference was the temperature during film growth.
Film (a) was grown at 488 K, whereas film (b)t was
grown at 573 K. Films grown at lower temperatures
are even worse than (a), and growth above 573 K
causes other problems. Thus, an optimum temperature
was obtained, along with a clear understanding of
the reason.
You can get a lot more information about this
subject on the internet. Here are a few search
engines
1. Alta Vista
2.
Google
3. Northern Light
4. Ask Jeeves
5. Infoseek
And here are a few good links to get you
started.
You can read more about STM's...
1.
2. 3. 4. 
From the manufacturers of STM's...
5. 6. 
And those guys at IBM really go all out...
7.
Research
Questions (1 point extra credit each!)
- Binnig and Rohrer shared their Nobel Prize
with a third scientist. Who was he, and what
did he do?
- The STM typically operates with a bias
voltage of a few volts. What is a typical
tunneling current?
- The STM tip must be moved with
extraordinary precision as it follows the
surface. What devices are used to accomplish
this?
- STM was the first, but there are now other
forms of "scanned probe microscopy". What are
they? How do they work?
This site is made possible by
funding from the National Science Foundation
(DUE-9981111). ©2001 A. Gavrin and G. Novak, all rights reserved.
|